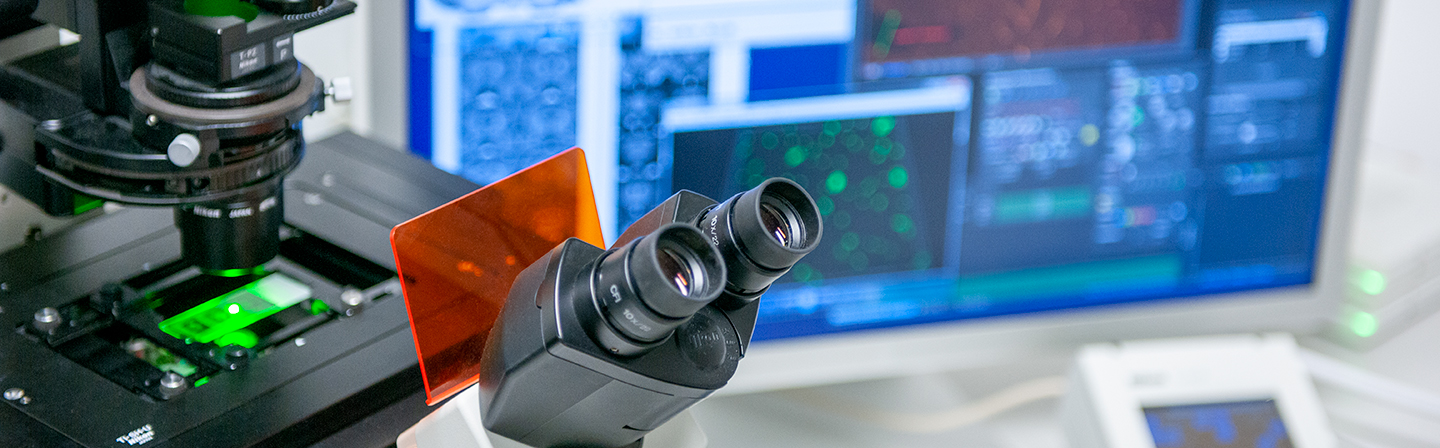
The field of life sciences presents us with a number of imaging methods. Procedures applied in this field draw on a broad span of the electromagnetic spectrum, ranging from short-wave roentgen radiation (computer tomography) to light that is visible to humans (microscopy), right over to the radio frequency range (magnetic resonance imaging). Each one of these procedures is able to pinpoint structures or biological processes in the living organism with great precision.
The unit is focusing here on developing imaging solutions to scientific tasks both with and for partners from the fields of research and industry. Besides pure data acquisition, projects up to device development are also implemented. In interdisciplinary consortia, the working group serves as an interface between engineering and life sciences.
The technologies available at the Fraunhofer IZI for experimental imaging include:
As part of an institute-wide network of bioanalytical methods, other techniques such as mass spectrometry, flow cytometry and DNA sequencing can be used to assess biological samples. Further information on this and a detailed equipment overview can be found on the homepage of the Advanced Analytics Technology Platform.