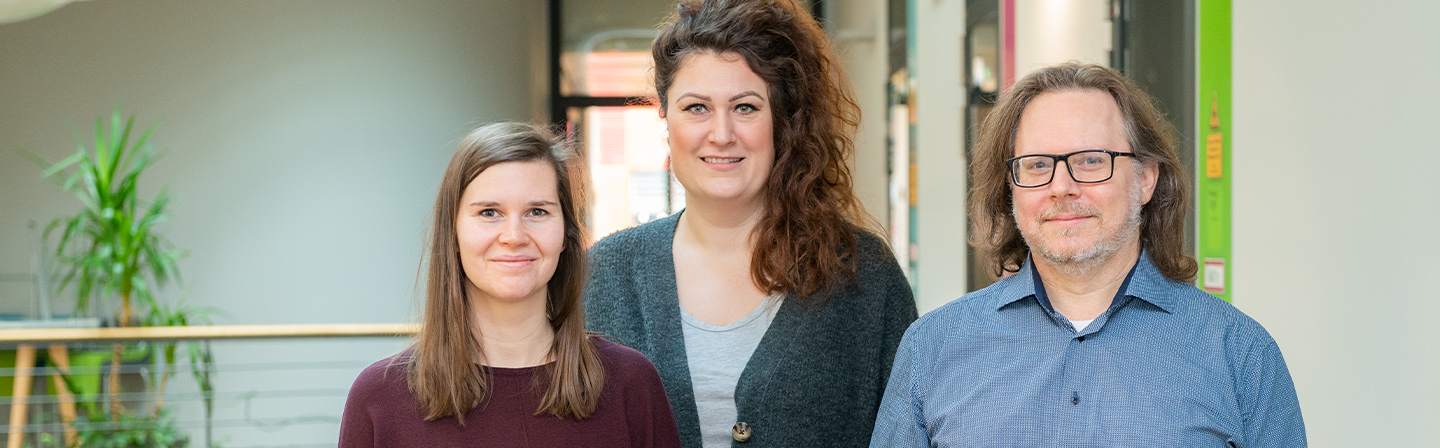
The work undertaken in this unit focuses on developing diagnostic and therapeutic applications of nanomaterials constructed by methods such as DNA self-assembly and molecular programming. Founded in 2013 as a part of the Fraunhofer Attract program, the unit’s aim is to develop concrete DNA-based tools for research and biomedicine, as well as to investigate and exploit the underlying material properties of nanoparticles built from DNA and composites. One aspect centers on the ability of DNA-based templates to serve as precise guides for the nanometer-scale arrangement of basic components for biosensors and nanocircuitry. Additionally, the unit develops functional platforms from DNA and other materials for the efficient transport of molecules in vitro and in vivo. Mechanical properties of DNA platforms as well as emergent properties from composites are also examined as possible instruments to increase the functional nature of hybrid materials.